Blogs
Top Blogs
1
Smoking, Methylation, and Cancer— The Importance of Epigenetic Changes
31 May 2025
Strand Content Team
2
HRD Testing: From Genomic Instability to Impactful Clinical Decision-Making
08 May 2025
Strand Content Team
3
Array vs NGS Technologies for Transgenic Crop Research in Agriculture
09 May 2025
Dr Harsha P.K
4
Improving Hereditary Cancer Detection with NGS + Digital MLPA
06 May 2025
Dr. Rakesh Sharma
Blogs
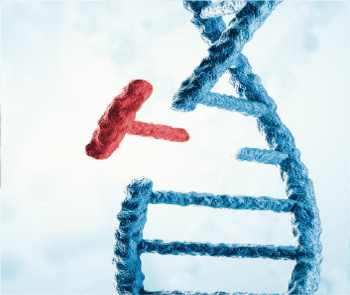
Unmasking Silent Threats: Genetic Mutations and Sudden Cardiac Events
A study from 2013 titled ‘Lives forever changed: Family bereavement experiences after sudden cardiac death’, offers a poignant perspective into the world of families shaken by such a loss [1]. The shock and confusion of seeing a vibrant, healthy, young family member collapse and pass away within an hour leaves many bereaved families haunted with doubts. Could they have possibly known? Could something have been done to prevent or prepare?
These touching accounts are a testimony to the great medical and psychosocial value of being familiar with one’s genetic predispositions, particularly concerning the heart. Advanced research is unearthing more and more each year about genes associated with fatal cardiac outcomes in individuals and families. The gene KCNQ1, on chromosome 11, is one of them.
The KCNQ1 gene encodes a protein that helps build ion channels transporting potassium ions (K+) across cells. Such channels are very important for biological processes that involve electrical signaling, such as heartbeats and nerve impulse transmission. Many types of K+ channels are present all over the body, each with its own electric current profile. The type that the KCNQ1 gene encodes is voltage sensitive, and is found particularly in the heart and the inner ear.
When the KCNQ1 protein is changed because of a gene mutation, the properties of the channel also get affected. The time and pattern of electric flow through the channels get altered, and heart rhythms become irregular. This leads to a plethora of heritable heart conditions which cause arrhythmia.
The most common of these is Long QT syndrome 1 (LQTS1), or Romano Ward syndrome, where the muscles of the lower chamber of the heart (ventricles) take longer than average to recharge between beats. The name ‘Long QT’ describes this delay, which appears in an electrocardiogram or EKG as a prolonged ‘QT’ interval. The prevalence of LQTS1 is estimated to be about 1 in 2000 in western countries [2], but the prevalence rate in India is not still clear.
During a long QT episode, usually triggered by physical or emotional stress, the ventricles start beating fast and chaotically to pump enough blood, a phenomenon known as ‘tachyarrhythmia’. Individuals can experience dizziness, blurred vision, palpitations, and heart rates that may go up to 300 beats per minute, compared to the normal 60-100, showing up in the EKG as many twisting peaks called ‘torsades des pointes’. This is a serious condition which disturbs proper flow of blood to the brain, leading to sudden fainting.
Usually the episode subsides and the heart rhythm normalizes on its own. However, if it continues, the heart muscles may start twitching uncoordinatedly. They may get overcome and stop pumping blood, leading to cardiac arrest and sudden death of a seemingly healthy individual. LQTS1 is also implicated in sudden infant death syndrome, and a small percentage of infants who pass away due to SIDS carry mutations in LQTS causing genes, including KCNQ1 [3].
In some cases, KCNQ1 related long QT syndrome may be associated with other symptoms such as congenital deafness. This condition is known as Jervell and Lange-Nielsen syndrome (JLNS). Less commonly, other kinds of arrhythmias may arise, including atrial fibrillation, which causes unsynchronized activity in the upper chambers of the heart, and short QT syndrome, characterized by an abnormally shortened QT interval.
Romano-Ward syndrome, the most common of long QT syndromes, is inherited in a dominant manner, which means only one mutated copy of the gene is sufficient to cause disease. In fact, studies have demonstrated that the mutated protein can bind to its normal, ‘wild-type’ versions in the body and act like a ‘poison-pill’, inhibiting their activity as well [4].
Mutations in KCNQ1 do not often occur spontaneously and are mostly inherited, and the extent to which clinical symptoms may or may not arise varies between individuals. JLNS, though, is recessively inherited, and is therefore rarer [5].
More than 100 mutations have been identified in KCNQ1, associated with LQTS1. Yet, everyone who carries a genetic variation may not develop symptoms. This phenomenon is called reduced ‘penetrance’, which means the proportion of people with the variation who go on to actually manifest symptoms is relatively low. Studies indicate that more than 50% of individuals who have a gene variation associated with LQTS do not show any clinical symptoms, and about 12 to 14% do not even show prolonged QTc intervals (QT intervals corrected for heart rate).
However, some people with genetic predisposition may start showing delayed QTc intervals in response to certain drugs or electrolyte imbalance. This is known as acquired long QT syndrome, and is usually temporary [5,6,7].
90% of those who eventually become symptomatic have usually had their first episode by the age of 40, sometimes even by the age of 12. Many symptomatic individuals experience episodes of fainting or dizziness without ever having a serious cardiac event. 1 to 3% of symptomatic individuals, however, do experience serious cardiac arrest or sudden cardiac death. Rarely, some may experience it as their first and only episode [5].
The variability with which the symptoms of LQTS1 show up is one of its most intriguing aspects, where even among members of the same family carrying the same mutations, some may face mild to no symptoms while others may have passed away due to sudden cardiac death. Recent efforts to understand why this is so has offered some possibilities, a plausible one being that changes in other genes interact with LQTS1 related variations, and act as modifiers that can either protect the person from developing symptoms or worsen them [8].
Early detection of KCNQ1 mutations can be very useful, even life saving. Once a person is found positive for a likely disease causing mutation in KCNQ1, doctors can plan the next steps of their care by looking at their individual and family medical histories. They may consider aspects such as – is the person asymptomatic as of now? Have they had unexplained fainting spells or sudden lightheadedness in early life? Have other family members complained of similar symptoms? Is there a history of sudden cardiac arrest, or sudden death at a young age in the family? Very often, doctors will recommend that family members, both immediate and extended, also get tested so that the penetrance of the particular mutation can be understood, all members at risk can receive preventive care as early as possible, and non gene carriers can be reassured.
Even in individuals with no symptoms, lifestyle changes are a must to ensure that there is no undue stress on the heart. Heavy exercise, smoking, drinking, fatty food should be ceased. Weight management and dental hygiene need to be ensured as well. Activities like swimming and driving that need constant alertness should be supervised or measured. Anxiety, shock, or deep emotional distress can trigger arrhythmic episodes, so a calm and regulated environment should be prioritized as much as possible. It is very important to routinely monitor one’s cardiac health through checkups and tests such as EKG. A combination of these preventive measures practiced in a regular, disciplined manner can be transformative in its effect on heart health.
Doctors may also start preventive medication based on the person’s health status. Beta blockers, which can regulate sudden spikes in heart rate and improve blood flow through vessels, are considered the primary line of treatment for LQTS1. Individuals with a KCNQ1 mutation and relevant family history who still show mild to no symptoms can be prescribed beta blockers, which have been associated with a drastic decrease in the rate of cardiac events – from 0.97 to 0.31 events per patient per year [5]. A study monitoring LQTS1 patients over a decade found that beta-blockers could bring down life-threatening cardiac events by as much as 97% [9].
Those who are already showing cardiac symptoms that are not responding to beta blockers, or have very high risk (two known disease-causing mutations) may be recommended a procedure called left cardiac sympathetic denervation (LCSD), which removes certain nerves that can cause irregular rhythms from the heart. LCSD has been shown to significantly reduce cardiac events over long term periods of up to 5 years [5].
People at a high risk for cardiac arrest or have survived one, are usually implanted with an implantable cardioverter-defibrillator (ICD), which is a small battery-powered device in the chest that can detect and correct irregular heartbeat through electric shocks.
Recent research seems to show promise in coming up with more effective treatment techniques. One such candidate is gene therapy, which aims to hit at the root of the problem by fixing the defective gene in the pulsatile tissues of the heart. Researchers successfully replaced the whole KCNQ1 gene in an in vitro heart tissue model, which restored KCNQ1 proteins to normal levels [10].
As more populations undergo genetic screening, more information about which variants or combinations of variants are associated with severe symptoms are being recorded. Taking genetic data together with multiple factors such as age, sex, autonomic control of nervous system etc, more targeted and personalized approaches can be created to combat KCNQ1 related disease or death.
Bibliography
- Rosenfeld, A. G., & Gilbert, K. (2013). Lives forever changed: family bereavement experiences after sudden cardiac death. Applied Nursing Research, 26(4), 168-173.
- Romano-Ward syndrome: MedlinePlus Genetics
- Modell, S. M., & Lehmann, M. H. (2006). The long QT syndrome family of cardiac ion channelopathies: a HuGE review. Genetics in Medicine, 8(3), 143-155.
- Herbert, E., Trusz-Gluza, M., Moric, E., Smilowska-Dzielicka, E., Mazurek, U., & Wilczok, T. (2002). KCNQ1 gene mutations and the respective genotype-phenotype correlations in the long QT syndrome. Medical Science Monitor, 8(10), RA240-RA248.
- Mizusawa, Y., Horie, M., & Wilde, A. A. (2014). Genetic and clinical advances in congenital long QT syndrome. Circulation Journal, 78(12), 2827-2833.
- Chen, S., Zhang, L., Bryant, R. M., Vincent, G. M., Flippin, M., Lee, J. C., … & Wang, Q. (2003). KCNQ1 mutations in patients with a family history of lethal cardiac arrhythmias and sudden death. Clinical genetics, 63(4), 273-282.
- Doolan, A., Langlois, N., Chiu, C., Ingles, J., Lind, J. M., & Semsarian, C. (2008). Postmortem molecular analysis of KCNQ1 and SCN5A genes in sudden unexplained death in young Australians. International journal of cardiology, 127(1), 138-141.
- Duchatelet, S., Crotti, L., Peat, R. A., Denjoy, I., Itoh, H., Berthet, M., … & Schwartz, P. J. (2013). Identification of a KCNQ1 polymorphism acting as a protective modifier against arrhythmic risk in long-QT syndrome. Circulation: Cardiovascular Genetics, 6(4), 354-361.
- Etheridge, S. P., Asaki, S. Y., & Niu, M. C. I. (2019). A personalized approach to long QT syndrome. Current Opinion in Cardiology, 34(1), 46-56.
- Dotzler, S. M., Kim, C. J., Gendron, W. A., Zhou, W., Ye, D., Bos, J. M., … & Ackerman, M. J. (2021). Suppression-replacement KCNQ1 gene therapy for type 1 long QT syndrome. Circulation, 143(14), 1411-1425.